A light-emitting diode (LED) is a semiconductor light source. LEDs are used as indicator lamps in many devices, and are increasingly used for lighting. Introduced as a practical electronic component in 1962, early LEDs emitted low-intensity red light, but modern versions are available across the visible, ultraviolet and infrared wavelengths, with very high brightness.
The LED is based on the semiconductor diode. When a diode is forward biased (switched on), electrons are able to recombine with holes within the device, releasing energy in the form of photons. This effect is called electroluminescence and the color of the light (corresponding to the energy of the photon) is determined by the energy gap of the semiconductor. An LED is usually small in area (less than 1 mm2), and integrated optical components are used to shape its radiation pattern and assist in reflection. LEDs present many advantages over incandescent light sources including lower energy consumption, longer lifetime, improved robustness, smaller size, faster switching, and greater durability and reliability. LEDs powerful enough for room lighting are relatively expensive and require more precise current and heat management than compact fluorescent lamp sources of comparable output.
They are used in applications as diverse as replacements for aviation lighting, automotive lighting (particularly indicators) and in traffic signals. The compact size of LEDs has allowed new text and video displays and sensors to be developed, while their high switching rates are useful in advanced communications technology. Infrared LEDs are also used in the remote control units of many commercial products including televisions, DVD players, and other domestic appliances.

Light-emitting diode

Electronic symbol
History
Discoveries and early devices
Electroluminescence was discovered in 1907 by the British experimenter H. J. Round of Marconi Labs, using a crystal of silicon carbide and a cat's-whisker detector. Russian Oleg Vladimirovich Losev independently reported on the creation of an LED in 1927. His research was distributed in Russian, German and British scientific journals, but no practical use was made of the discovery for several decades. Rubin Braunstein of the Radio Corporation of America reported on infrared emission from gallium arsenide (GaAs) and other semiconductor alloys in 1955. Braunstein observed infrared emission generated by simple diode structures using gallium antimonide (GaSb), GaAs, indium phosphide (InP), and silicon-germanium (SiGe) alloys at room temperature and at 77 kelvin.
In 1961, American experimenters Robert Biard and Gary Pittman working at Texas Instruments, found that GaAs emitted infrared radiation when electric current was applied and received the patent for the infrared LED.
The first practical visible-spectrum (red) LED was developed in 1962 by Nick Holonyak Jr., while working at General Electric Company. Holonyak is seen as the "father of the light-emitting diode". M. George Craford, a former graduate student of Holonyak, invented the first yellow LED and improved the brightness of red and red-orange LEDs by a factor of ten in 1972. In 1976, T.P. Pearsall created the first high-brightness, high efficiency LEDs for optical fiber telecommunications by inventing new semiconductor materials specifically adapted to optical fiber transmission wavelengths.
Up to 1968 visible and infrared LEDs were extremely costly, on the order of US $200 per unit, and so had little practical application. The Monsanto Company was the first organization to mass-produce visible LEDs, using gallium arsenide phosphide in 1968 to produce red LEDs suitable for indicators. Hewlett Packard (HP) introduced LEDs in 1968, initially using GaAsP supplied by Monsanto. The technology proved to have major applications for alphanumeric displays and was integrated into HP's early handheld calculators. In the 1970s commercially successful LED devices at under five cents each were produced by Fairchild Optoelectronics. These devices employed compound semiconductor chips fabricated with the planar process invented by Dr. Jean Hoerni at Fairchild Semiconductor. The combination of planar processing for chip fabrication and innovative packaging techniques enabled the team at Fairchild led by optoelectronics pioneer Thomas Brandt to achieve the necessary cost reductions. These techniques continue to be used by LED producers.
Practical use
he first commercial LEDs were commonly used as replacements for incandescent and neon indicator lamps, and in seven-segment displays, first in expensive equipment such as laboratory and electronics test equipment, then later in such appliances as TVs, radios, telephones, calculators, and even watches. These red LEDs were bright enough only for use as indicators, as the light output was not enough to illuminate an area. Readouts in calculators were so small that plastic lenses were built over each digit to make them legible. Later, other colors became widely available and also appeared in appliances and equipment. As the LED materials technology became more advanced, the light output was increased, while maintaining the efficiency and the reliability to an acceptable level. The invention and development of the high power white light LED led to use for illumination. Most LEDs were made in the very common 5 mm T1¾ and 3 mm T1 packages, but with increasing power output, it has become increasingly necessary to shed excess heat in order to maintain reliability, so more complex packages have been adapted for efficient heat dissipation. Packages for state-of-the-art high power LEDs bear little resemblance to early LEDs.
Continuing development
The first high-brightness blue LED was demonstrated by Shuji Nakamura of Nichia Corporation and was based on InGaN borrowing on critical developments in GaN nucleation on sapphire substrates and the demonstration of p-type doping of GaN which were developed by Isamu Akasaki and H. Amano in Nagoya. In 1995, Alberto Barbieri at the Cardiff University Laboratory (GB) investigated the efficiency and reliability of high-brightness LEDs and demonstrated a very impressive result by using a transparent contact made of indium tin oxide (ITO) on (AlGaInP/GaAs) LED. The existence of blue LEDs and high efficiency LEDs quickly led to the development of the first white LED, which employed a Y3Al5O12:Ce, or "YAG", phosphor coating to mix yellow (down-converted) light with blue to produce light that appears white. Nakamura was awarded the 2006 Millennium Technology Prize for his invention.
The development of LED technology has caused their efficiency and light output to increase exponentially, with a doubling occurring about every 36 months since the 1960s, in a way similar to Moore's law. The advances are generally attributed to the parallel development of other semiconductor technologies and advances in optics and material science. This trend is normally called Haitz's Law after Dr. Roland Haitz.
In February 2008, Bilkent university in Turkey reported 300 lumens of visible light per watt luminous efficacy (not per electrical watt) and warm light by using nanocrystals.
In January 2009, researchers from Cambridge University reported a process for growing gallium nitride (GaN) LEDs on silicon. Production costs could be reduced by 90% using six-inch silicon wafers instead of two-inch sapphire wafers. The team was led by Colin Humphreys.
Technology
Physics
Like a normal diode, the LED consists of a chip of semiconducting material doped with impurities to create a p-n junction. As in other diodes, current flows easily from the p-side, or anode, to the n-side, or cathode, but not in the reverse direction. Charge-carriers—electrons and holes—flow into the junction from electrodes with different voltages. When an electron meets a hole, it falls into a lower energy level, and releases energy in the form of a photon.
The wavelength of the light emitted, and therefore its color, depends on the band gap energy of the materials forming the p-n junction. In silicon or germanium diodes, the electrons and holes recombine by a non-radiative transition which produces no optical emission, because these are indirect band gap materials. The materials used for the LED have a direct band gap with energies corresponding to near-infrared, visible or near-ultraviolet light.
LED development began with infrared and red devices made with gallium arsenide. Advances in materials science have made possible the production of devices with ever-shorter wavelengths, producing light in a variety of colors.
LEDs are usually built on an n-type substrate, with an electrode attached to the p-type layer deposited on its surface. P-type substrates, while less common, occur as well. Many commercial LEDs, especially GaN/InGaN, also use sapphire substrate.
Most materials used for LED production have very high refractive indices. This means that much light will be reflected back into the material at the material/air surface interface. Therefore Light extraction in LEDs is an important aspect of LED production, subject to much research and development.

Parts of an LED
Efficiency and operational parameters
Typical indicator LEDs are designed to operate with no more than 30–60 milliwatts [mW] of electrical power. Around 1999, Philips Lumileds introduced power LEDs capable of continuous use at one watt [W]. These LEDs used much larger semiconductor die sizes to handle the large power inputs. Also, the semiconductor dies were mounted onto metal slugs to allow for heat removal from the LED die.
One of the key advantages of LED-based lighting is its high efficiency, as measured by its light output per unit power input. White LEDs quickly matched and overtook the efficiency of standard incandescent lighting systems. In 2002, Lumileds made five-watt LEDs available with a luminous efficacy of 18–22 lumens per watt [lm/W]. For comparison, a conventional 60–100 W incandescent lightbulb produces around 15 lm/W, and standard fluorescent lights produce up to 100 lm/W. A recurring problem is that efficiency will fall dramatically for increased current. This effect is known as droop and effectively limits the light output of a given LED, increasing heating more than light output for increased current.
In September 2003, a new type of blue LED was demonstrated by the company Cree, Inc. to provide 24 mW at 20 milliamperes [mA]. This produced a commercially packaged white light giving 65 lm/W at 20 mA, becoming the brightest white LED commercially available at the time, and more than four times as efficient as standard incandescents. In 2006 they demonstrated a prototype with a record white LED luminous efficacy of 131 lm/W at 20 mA. Also, Seoul Semiconductor has plans for 135 lm/W by 2007 and 145 lm/W by 2008, which would be approaching an order of magnitude improvement over standard incandescents and better even than standard fluorescents. Nichia Corporation has developed a white LED with luminous efficacy of 150 lm/W at a forward current of 20 mA.
High-power (≥ 1 W) LEDs are necessary for practical general lighting applications. Typical operating currents for these devices begin at 350 mA.
Note that these efficiencies are for the LED chip only, held at low temperature in a lab. In a lighting application, operating at higher temperature and with drive circuit losses, efficiencies are much lower. United States Department of Energy (DOE) testing of commercial LED lamps designed to replace incandescent lamps or CFLs showed that average efficacy was still about 46 lm/W in 2009 (tested performance ranged from 17 lm/W to 79 lm/W).
Cree issued a press release on February 3, 2010 about a laboratory prototype LED achieving 208 lumens per watt at room temperature. The correlated color temperature was reported to be 4579 K
The inner workings of an LED
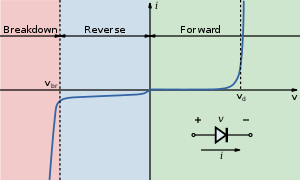
I-V diagram for a diode an LED will begin to emit light when the on-voltage is exceeded. Typical on voltages are 2-3 Volt
Lifetime and failure
Solid state devices such as LEDs are subject to very limited wear and tear if operated at low currents and at low temperatures. Many of the LEDs produced in the 1970s and 1980s are still in service today. Typical lifetimes quoted are 25,000 to 100,000 hours but heat and current settings can extend or shorten this time significantly.
The most common symptom of LED (and diode laser) failure is the gradual lowering of light output and loss of efficiency. Sudden failures, although rare, can occur as well. Early red LEDs were notable for their short lifetime. With the development of high-power LEDs the devices are subjected to higher junction temperatures and higher current densities than traditional devices. This causes stress on the material and may cause early light output degradation. To quantitatively classify lifetime in a standardized manner it has been suggested to use the terms L75 and L50 which is the time it will take a given LED to reach 75% and 50% light output respectively.
Like other lighting devices, LED performance is temperature dependent. Most manufacturers' published ratings of LEDs are for an operating temperature of 25°C. LEDs used outdoors, such as traffic signals or in-pavement signal lights, and that are utilized in climates where the temperature within the luminaire gets very hot, could result in low signal intensities or even failure.
LEDs maintain consistent light output even in cold temperatures, unlike traditional lighting methods. Consequently, LED technology may be a good replacement in areas such as supermarket freezer lighting and will last longer than other technologies. Because LEDs do not generate as much heat as incandescent bulbs, they are an energy-efficient technology to use in such applications such as freezers. On the other hand, because they do not generate much heat, ice and snow may build up on the LED luminaire in colder climates. This has been a problem plaguing airport runway lighting, although some research has been done to try to develop heat sink technologies in order to transfer heat to alternative areas of the luminaire.
Colors and materials
Color | Wavelength (nm) | Voltage (V) | Semiconductor Material | |
Infrared | λ > 760 | ΔV < 1.9 | Gallium arsenide (GaAs) Aluminium gallium arsenide (AlGaAs) | |
Red | 610 < λ < 760 | 1.63 < ΔV < 2.03 | Aluminium gallium arsenide (AlGaAs) Gallium arsenide phosphide (GaAsP) Aluminium gallium indium phosphide (AlGaInP) Gallium(III) phosphide (GaP) | |
Orange | 590 < λ < 610 | 2.03 < ΔV < 2.10 | Gallium arsenide phosphide (GaAsP) Aluminium gallium indium phosphide (AlGaInP) Gallium(III) phosphide (GaP) | |
Yellow | 570 < λ < 590 | 2.10 < ΔV < 2.18 | Gallium arsenide phosphide (GaAsP) Aluminium gallium indium phosphide (AlGaInP) Gallium(III) phosphide (GaP) | |
Green | 500 < λ < 570 | 1.9 < ΔV < 4.0 | Indium gallium nitride (InGaN) / Gallium(III) nitride (GaN) Gallium(III) phosphide (GaP) Aluminium gallium indium phosphide (AlGaInP) Aluminium gallium phosphide (AlGaP) | |
Blue | 450 < λ < 500 | 2.48 < ΔV < 3.7 | Zinc selenide (ZnSe) Indium gallium nitride (InGaN) Silicon carbide (SiC) as substrate Silicon (Si) as substrate — (under development) | |
Violet | 400 < λ < 450 | 2.76 < ΔV < 4.0 | Indium gallium nitride (InGaN) | |
Purple | multiple types | 2.48 < ΔV < 3.7 | Dual blue/red LEDs, blue with red phosphor, or white with purple plastic | |
Ultraviolet | λ < 400 | 3.1 < ΔV < 4.4 | Diamond (235 nm) Boron nitride (215 nm) Aluminium nitride (AlN) (210 nm) Aluminium gallium nitride (AlGaN) Aluminium gallium indium nitride (AlGaInN) (down to 210 nm) | |
White | Broad spectrum | ΔV = 3.5 | Blue/UV diode with yellow phosphor |
Types

LEDs are produced in a variety of shapes and sizes. The 5 mm cylindrical package (red, fifth from the left) is the most common, estimated at 80% of world production. The color of the plastic lens is often the same as the actual color of light emitted, but not always. For instance, purple plastic is often used for infrared LEDs, and most blue devices have clear housings. There are also LEDs in SMT packages, such as those found on blinkies and on cell phone keypads (not shown).
Miniature LEDs
These are mostly single-die LEDs used as indicators, and they come in various-sizes from 2 mm to 8 mm, through-hole and surface mount packages. They are usually simple in design, not requiring any separate cooling body.[67] Typical current ratings ranges from around 1 mA to above 20 mA. The small scale sets a natural upper boundary on power consumption due to heat caused by the high current density and need for heat sinking.
Different sized LEDs. 8 mm, 5 mm and 3 mm, with a wooden match-stick for scale.
High power LEDs
High power LEDs (HPLED) can be driven at currents from hundreds of mA to more than an ampere, compared with the tens of mA for other LEDs. Some can produce over a thousand [68][69] lumens. Since overheating is destructive, the HPLEDs must be mounted on a heat sink to allow for heat dissipation. If the heat from a HPLED is not removed, the device will burn out in seconds. A single HPLED can often replace an incandescent bulb in a torch, or be set in an array to form a powerful LED lamp.
Some well-known HPLEDs in this category are the Lumileds Rebel Led, Osram Opto Semiconductors Golden Dragon and Cree X-lamp. As of September 2009 some HPLEDs manufactured by Cree Inc. now exceed 105 lm/W [70] (e.g. the XLamp XP-G LED chip emitting Cool White light) and are being sold in lamps intended to replace incandescent, halogen, and even fluorescent style lights as LEDs become more cost competitive.
LEDs have been developed by Seoul Semiconductor that can operate on AC power without the need for a DC converter. For each half cycle part of the LED emits light and part is dark, and this is reversed during the next half cycle. The efficacy of this type of HPLED is typically 40 lm/W.[71] A large number of LED elements in series may be able to operate directly from line voltage. In 2009 Seoul Semiconductor released a high DC voltage capable of being driven from AC power with a simple controlling circuit. The low power dissipation of these LEDs affords them more flexibility than the original AC LED design.

High-power light emiting diodes
Mid-range LEDs
Medium power LEDs are often through-hole mounted and used when an output of a few lumen is needed. They sometimes have the diode mounted to four leads (two cathode leads, two anode leads) for better heat conduction and carry an integrated lens. An example of this is the Superflux package, from Philips Lumileds. These LEDs are most commonly used in light panels, emergency lighting and automotive tail-lights. Due to the larger amount of metal in the LED, they are able to handle higher currents (around 100 mA). The higher current allows for the higher light output required for tail-lights and emergency lighting.
Application-specific variations
Flashing LEDs are used as attention seeking indicators without requiring external electronics. Flashing LEDs resemble standard LEDs but they contain an integrated multivibrator circuit which causes the LED to flash with a typical period of one second. In diffused lens LEDs this is visible as a small black dot. Most flashing LEDs emit light of a single color, but more sophisticated devices can flash between multiple colors and even fade through a color sequence using RGB color mixing.
Bi-color LEDs are actually two different LEDs in one case. They consist of two dies connected to the same two leads antiparallel to each other. Current flow in one direction produces one color, and current in the opposite direction produces the other color. Alternating the two colors with sufficient frequency causes the appearance of a blended third color. For example, a red/green LED operated in this fashion will color blend to produce a yellow appearance.
Tri-color LEDs are two LEDs in one case, but the two LEDs are connected to separate leads so that the two LEDs can be controlled independently and lit simultaneously. A three-lead arrangement is typical with one common lead (anode or cathode).
RGB LEDs contain red, green and blue emitters, generally using a four-wire connection with one common lead (anode or cathode). These LEDs can have either common positive or common negative leads. Others however, have only two leads (positive and negative) and have a built in tiny electronic control unit.
Alphanumeric LED displays are available in seven-segment and starburst format. Seven-segment displays handle all numbers and a limited set of letters. Starburst displays can display all letters. Seven-segment LED displays were in widespread use in the 1970s and 1980s, but increasing use of liquid crystal displays, with their lower power consumption and greater display flexibility, has reduced the popularity of numeric and alphanumeric LED displays.
Considerations for use
Power sources
The current/voltage characteristic of an LED is similar to other diodes, in that the current is dependent exponentially on the voltage (see Shockley diode equation). This means that a small change in voltage can lead to a large change in current. If the maximum voltage rating is exceeded by a small amount the current rating may be exceeded by a large amount, potentially damaging or destroying the LED. The typical solution is therefore to use constant current power supplies, or driving the LED at a voltage much below the maximum rating. Since most household power sources (batteries, mains) are not constant current sources, most LED fixtures must include a power converter. However, the I/V curve of nitride-based LEDs is quite steep above the knee and gives an If of a few milliamperes at a Vf of 3 V, making it possible to power a nitride-based LED from a 3 V battery such as a coin cell without the need for a current limiting resistor.
Electrical polarity
As with all diodes, current flows easily from p-type to n-type material. However, no current flows and no light is produced if a small voltage is applied in the reverse direction. If the reverse voltage becomes large enough to exceed the breakdown voltage, a large current flows and the LED may be damaged. If the reverse current is sufficiently limited to avoid damage, the reverse-conducting LED is a useful noise diode.
Safety
The vast majority of devices containing LEDs are "safe under all conditions of normal use", and so are classified as "Class 1 LED product"/"LED Klasse 1". At present, only a few LEDs—extremely bright LEDs that also have a tightly focused viewing angle of 8° or less—could, in theory, cause temporary blindness, and so are classified as "Class 2". In general, laser safety regulations—and the "Class 1", "Class 2", etc. system—also apply to LEDs.
Advantages
-Efficiency: LEDs produce more light per watt than incandescent bulbs.[75] Their efficiency is not affected by shape and size, unlike Fluorescent light bulbs or tubes.
-Color: LEDs can emit light of an intended color without the use of the color filters that traditional lighting methods require. This is more efficient and can lower initial costs.
-Size: LEDs can be very small (smaller than 2 mm2) and are easily populated onto printed circuit boards.
-On/Off time: LEDs light up very quickly. A typical red indicator LED will achieve full brightness in under a microsecond. LEDs used in communications devices can have even faster response times.
-Cycling: LEDs are ideal for use in applications that are subject to frequent on-off cycling, unlike fluorescent lamps that burn out more quickly when cycled frequently, or HID lamps that require a long time before restarting.
-Dimming: LEDs can very easily be dimmed either by pulse-width modulation or lowering the forward current.
-Cool light: In contrast to most light sources, LEDs radiate very little heat in the form of IR that can cause damage to sensitive objects or fabrics. Wasted energy is dispersed as heat through the base of the LED.
-Slow failure: LEDs mostly fail by dimming over time, rather than the abrupt burn-out of incandescent bulbs.
-Lifetime: LEDs can have a relatively long useful life. One report estimates 35,000 to 50,000 hours of useful life, though time to complete failure may be longer. Fluorescent tubes typically are rated at about 10,000 to 15,000 hours, depending partly on the conditions of use, and incandescent light bulbs at 1,000–2,000 hours.
-Shock resistance: LEDs, being solid state components, are difficult to damage with external shock, unlike fluorescent and incandescent bulbs which are fragile.
-Focus: The solid package of the LED can be designed to focus its light. Incandescent and fluorescent sources often require an external reflector to collect light and direct it in a usable manner.
-Toxicity: LEDs do not contain mercury, unlike fluorescent lamps.
Disadvantages
-Some Fluorescent lamps can be more efficient.
-High initial price: LEDs are currently more expensive, price per lumen, on an initial capital cost basis, than most conventional lighting technologies. The additional expense partially stems from the relatively low lumen output and the drive circuitry and power supplies needed.
-Temperature dependence: LED performance largely depends on the ambient temperature of the operating environment. Over-driving the LED in high ambient temperatures may result in overheating of the LED package, eventually leading to device failure. Adequate heat-sinking is required to maintain long life. This is especially important when considering automotive, medical, and military applications where the device must operate over a large range of temperatures, and is required to have a low failure rate.
-Voltage sensitivity: LEDs must be supplied with the voltage above the threshold and a current below the rating. This can involve series resistors or current-regulated power supplies.
-Light quality: Most cool-white LEDs have spectra that differ significantly from a black body radiator like the sun or an incandescent light. The spike at 460 nm and dip at 500 nm can cause the color of objects to be perceived differently under cool-white LED illumination than sunlight or incandescent sources, due to metamerism, red surfaces being rendered particularly badly by typical phosphor based cool-white LEDs. However, the color rendering properties of common fluorescent lamps are often inferior to what is now available in state-of-art white LEDs.
-Area light source: LEDs do not approximate a "point source" of light, but rather a lambertian distribution. So LEDs are difficult to use in applications requiring a spherical light field. LEDs are not capable of providing divergence below a few degrees. This is contrasted with lasers, which can produce beams with divergences of 0.2 degrees or less.
-Blue hazard: There is a concern that blue LEDs and cool-white LEDs are now capable of exceeding safe limits of the so-called blue-light hazard as defined in eye safety specifications such as ANSI/IESNA RP-27.1-05: Recommended Practice for Photobiological Safety for Lamp and Lamp Systems.
-Blue pollution: Because cool-white LEDs, emit proportionally more blue light than conventional outdoor light sources such as high-pressure sodium lamps, the strong wavelength dependence of Rayleigh scattering means that cool-white LEDs can cause more light pollution than other light sources. The International Dark-Sky Association discourages the use of white light sources with correlated color temperature above 3,000 K.
Applications
-Application of LEDs fall into four major categories:
-Visual signal application where the light goes more or less directly from the LED to the human eye, to convey a message or meaning.
-Illumination where LED light is reflected from object to give visual response of these objects.
-Generate light for measuring and interacting with processes that do not involve the human visual system.
-Narrow band light sensors where the LED is operated in a reverse-bias mode and is responsive to incident light instead of emitting light.
Freddy Vallenilla, EES SECC 2
No hay comentarios:
Publicar un comentario